By Jennifer Heathcote, business development mgr., GEW, Inc.
Editor’s Note: This article won the AIMCAL Women’s Leadership Committee Best Paper Award at the 2020 AIMCAL R2R Virtual Conference USA, held online in October 2020.
Introduction
Ultraviolet (UV) curing systems are routinely integrated into roll-to-roll (R2R) and roll-to-sheet (R2S) manufacturing processes. This is because UV curing enables narrow-, mid-, and wide-web converters to rapidly set inks, coatings, adhesives, and extrusions inline, in a small footprint, and at high speed while also producing performance properties superior to what is achievable with conventionally dried materials. This is possible because UV curing is not drying. Instead, it is a molecular-level chemical reaction that transforms liquid-like materials that are wet-to-the touch into crosslinked polymers that are fully dry-to the touch. To the benefit of converters, this transformation occurs within a fraction of a second provided UV formulations are exposed to appropriate wavelengths of UV light between 100 and 450 nm and the total delivered energy is matched to the intended web speed.
UV-initiated reactions create strong chemical bonds between molecules; whereas, conventional drying processes leave disconnected residual solids resting on the surface of non-porous substrates, such as polymer films and coated papers, or dispersed within top layers of porous materials, such as uncoated papers. UV-initiated crosslinking processes naturally generate long, continuous molecular chains that drive highly desirable and robust functional and aesthetic properties. UV formulations are typically 100% solids, contain no liquid carriers that must be evaporated, and require no energy-consuming thermal dryers. Once a web exits a UV-curing station, it is immediately ready for further processing, sheeting, slitting, rewinding, and shipping. In addition, UV-cured surfaces do not scratch, mar, or become damaged when passed through downstream manufacturing-line components or finishing equipment. This keeps work-in-progress goods out of inventory, reduces scrap, and facilitates shorter lead times.
Mercury-vapor, light emitting diode (LED), and excimer lamps all deliver UV energy to webs in production environments. Some manufacturing processes incorporate combinations of the technologies to achieve specific properties not possible with one single technology on its own. Of the three, LEDs are the newest UV-emitting source with small, low-power prototypes first appearing on the market around 2005. The technology has evolved steadily over the last 15 years becoming more powerful, more capable, and more economical with each passing year. While UV-LED curing was first embraced by narrow-web converters nearly a decade ago, it is now viable for high-speed, wide-web processes. A few formulation holdouts still need to be fully developed for LED; however, the industry continues making progress and will introduce a growing number of LED solutions to the converting market over the coming years. This paper is meant to discuss the mechanics of UV-curing sources, applications that are feasible for UV-LED curing today along with what is still in development, and the path forward in UV-LED web converting.
UV curing
UV curing is a versatile technology employed across a range of printing and coating applications. It is suitable for inkjet, flexographic, gravure, screen, offset, slot-die, and Mayer-rod, among many other transfer and deposition methods. Examples of various types of UV-curing applications used by web converters is summarized in Table 1. Converters rely on UV curing for each of these applications because the technology delivers solidly reliable solutions for the numerous converting needs highlighted in Table 2.
TABLE 1. UV-curing applications employed by web converters
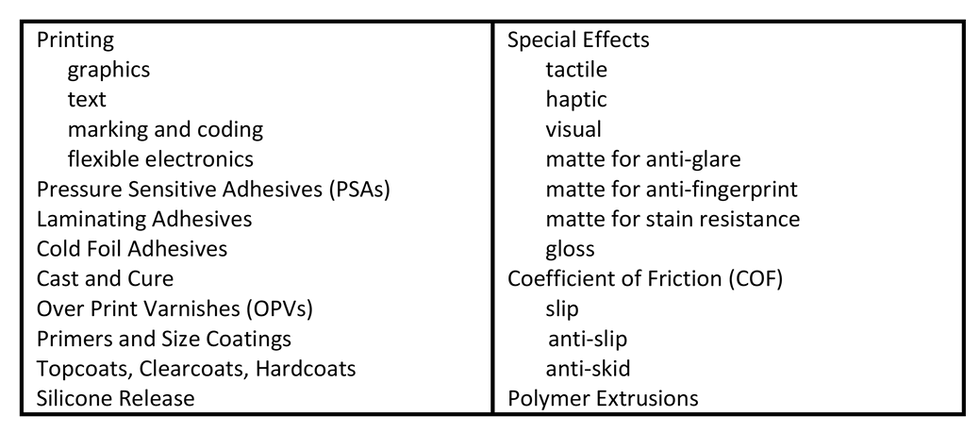
TABLE 2. Operation, economic, performance, and sustainability needs solved with UV curing
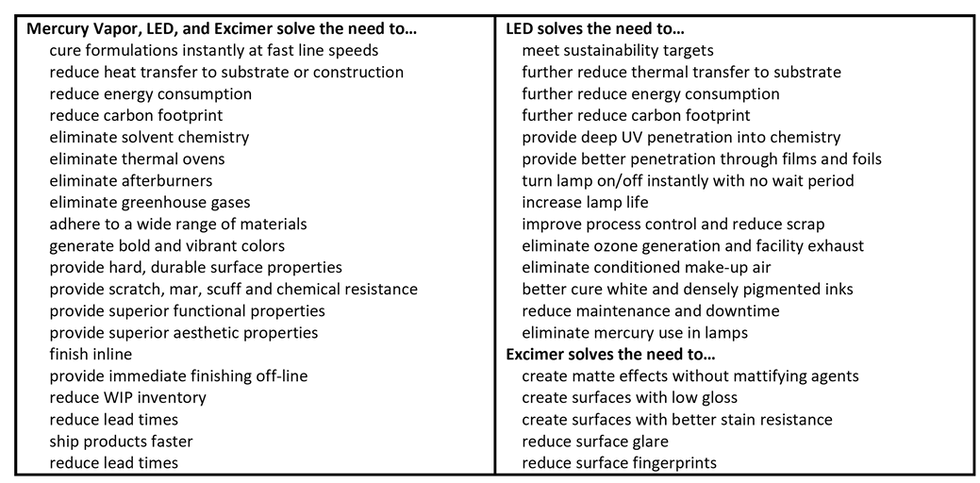
UV-curing sources for industrial applications
While mercury-vapor, LED, and excimer lamp technologies all emit ultraviolet energy, the mechanisms that generate the UV energy as well as the characteristics of the corresponding UV output are very different. Understanding these differences is instrumental in application and formulation development, UV-curing source selection, and process and manufacturing line integration.
Mercury-vapor lamps: Both electrode arc lamps and electrodeless microwave lamps fall within the category of mercury vapor. Mercury-vapor lamps are a type of medium-pressure, gas-discharge lamp in which a small amount of elemental mercury and a specific mix of inert gas are vaporized into a plasma within a sealed quartz tube. Plasma is an incredibly high-temperature ionized gas capable of conducting electricity. It is produced by applying an electrical voltage between two electrodes within an arc lamp or by microwaving an electrodeless lamp placed inside an enclosure.
Once vaporized, mercury plasma generates broad-spectrum UV output that radiates 360° from the quartz tube. Elliptical and parabolic reflectors located behind the quartz tube are used to concentrate the emitted UV energy onto the web. UV-curing systems using mercury-vapor lamps are cooled with forced air and/or circulated liquid. Mercury-vapor lamps have a short warm-up and cool-down period that occurs each time they are switched on or off. An image of several mercury-arc lamps and a lamphead assembly is shown in Figure 1 (a).
UV-LED lamps: LED systems are solid-state electronics composed of numerous chips of thin, semi-conductive, crystalline materials electrically wired together in a single row or a combination of rows and columns. In the case of UV LEDs, precisely designed and fabricated gallium nitride (GaN) and aluminum, gallium, nitride (AlGaN) materials emit relatively narrow-wavelength bands of ultraviolet energy when connected to a DC power source. The emitted light has a peak output centered at 365, 385, 395, or 405 nm, is projected forward from each LED a full 180°, is quickly and easily turned on or off, and has full linear adjustment of power.
Each LED is referred to as a positive-negative junction (p-n junction). This means that one portion of the LED has a positive charge, referred to as the anode (+). The other portion has a negative charge, referred to as the cathode (-). While both sides are relatively conductive, the junction boundary where the two sides meet, known as the depletion zone, is not conductive. When the positive (+) terminal of a direct-current (DC) power source is connected to the anode (+) of the LED, and the negative (-) terminal of the source is connected to the cathode (-), negatively charged electrons in the cathode and positively charged electron vacancies in the anode are repelled by the power source and pushed toward the mating depletion zone. This forward bias has the effect of overcoming the non-conductive boundary. When this occurs, free electrons in the n-type region cross over and fill vacancies in the p-type region. As electrons flow across the boundary, they transition to a state of lower energy. The respective drop in energy is released from the semiconductor as a combination of UV photons and heat. Typically, up to 40% of the differential is emitted as photons with electrical inefficiencies converting the balance into waste heat that must be removed from the system.
An illustration of three UV-LED modules integrated into a much longer array with numerous modules as well as a corresponding LED lamphead is shown in Figure 1 (b). Each of the purple squares in the graphic represents a single LED. Engineers package and power LEDs to emit the desired UV output for the intended market segment and application. Lamphead assemblies are then cooled with forced air or circulated liquid to maintain the necessary device operating temperate, remove waste heat, and ensure performance longevity.
Excimer lamps: Like mercury-vapor lamps, excimer lamps are a type of gas-discharge lamp. Unlike broadband mercury-vapor lamps, however, excimer lamps emit quasi-monochromatic ultraviolet energy without the use of elemental mercury. Excimer lamps are commonly available with ultraviolet outputs centered at 172, 222, 308, and 351 nm. Excimer lamps at 172 nm fall between 100-200 nm which is known as vacuum UV (VUV). The VUV band represents the shortest wavelengths of ultraviolet light. These wavelengths are absorbed by oxygen and, therefore, do not travel far through air. Ozone also is generated when wavelengths of 240 nm and less are absorbed by oxygen molecules.
Photons of vacuum-UV contain more energy than those of longer UV wavelengths but are only effective at curing the very top surface of UV formulations. In fact, 172-nm wavelengths are completely absorbed within the top 10 to 200 nm of UV-formulated chemistry. As a result, 172-nm excimer lamps crosslink only the outermost surface of UV formulations and must always be integrated in combination with mercury or LED systems to achieve full depth of cure. It is important to note that because vacuum-UV wavelengths are absorbed by air and incredibly efficient at generating ozone, 172-nm excimer lamps are always operated in a nitrogen-inerted environment when used for curing. Alternatively, the ozone produced by excimer radiation can be used to clean and sterilize surfaces in the absence of nitrogen. For these applications, ozone is a desirable by-product and introducing nitrogen decreases its concentration.
Excimer lamps consist of a quartz tube which serves as a dielectric barrier. The tube is filled with rare gases capable of forming excimer or exciplex molecules. Different gases produce different excited molecules and determine which specific wavelengths are emitted by the lamp. A coiled electrode runs along the inside length of the quartz tube while ground electrodes run along the outside length. Voltages are pulsed into the lamp at high frequencies. This causes electrons to flow within the internal electrode and discharge across the gas mixture toward the external ground electrodes. This scientific phenomenon is known as dielectric barrier discharge (DBD). As electrons travel through the gas, they interact with atoms and create energized or ionized species that produce excimer or exciplex molecules. Excimer and exciplex molecules have an incredibly short life, and as they decompose from an excited state to a ground state, photons of a quasi-monochromatic distribution are emitted. Excimer lamps generate negligible heat and require little if any lamphead-assembly cooling. An image of an excimer lamp and corresponding lamp head is shown in Figure 1 (c).
Critical differences in emitted wavelengths: One of the most significant differentiators of electrode arc, LED, and excimer lamps is spectral distribution. Mercury-vapor lamps are broadband in that they emit a mix of UVC (200 to 285 nm), UVB (285 to 315 nm), UVA (315 to 400 nm), UVV (400 to 450 nm), visible (400 to 700 nm), and infrared (700 nm to 1 mm) wavelengths. While radiated light of any wavelength contains energy that can be converted to heat, infrared wavelengths are the main thermal generating band. Today’s LEDs are commercially limited to UVA (365, 385, 395 nm) and UVV (405 nm) and contain no infrared. Excimer lamps peaking at 172 nm and used primarily for matte effects emit wavelengths entirely within the vacuum-UV (100 to 200 nm) band.
Shorter wavelengths have relatively reduced penetration coupled with relatively greater energy per photon. By contrast, longer wavelengths have greater penetration but contain less energy per photon. The relationship between wavelength absorption and depth of penetration for each ultraviolet band of energy is shown in Figure 2. While each photoinitiator reacts to a range of UV wavelengths, not all photoinitiators react to all wavelengths between 100 and 450 nm. Based on the laws of physics and availability of raw materials, formulators are tasked with sourcing and blending ingredients specifically designed to react to the unique wavelengths emitted by the lamp source while simultaneous delivering the desired final-cure properties at the intended web speed.
Transitioning to UV-LED web converting
Converters looking to transition to UV-LED curing and realize the long list of operation, economic, performance, and sustainability needs listed in Table 2 should consider several factors. This includes availability of LED-formulated chemistry for the intended application and transfer method, LED output matched to the requirements of the formulation and web speed, and proper and complete integration of the LED-curing system into the manufacturing line. Integration generally includes power, controls, cooling, and mounting as well as ancillary equipment, such as chilled rollers, chilled plates, web slots, nitrogen-inertion, shielding, adjustable lamp positioning, and the ability to move the lamphead assembly away from the web.

FIGURE 1. UV-curing lamps for R2R and R2S converting consist of three main types supplied in a wide range of lengths, spectral distributions, and power outputs.

FIGURE 2. Wavelengths of VUV and UVC are absorbed at the surface while wavelengths of UVA and UVV are absorbed throughout the formulation thickness. Formulations are designed to react to specific wavelength distributions emitted by UV-curing sources.
Today, UV-LED curing is a viable alternative for many ink, coating, and adhesive processes that currently use mercury-vapor lamps as well as conventional processes looking to embrace UV technology for the first time. This includes: 1) flexography, offset, gravure, screen, and inkjet inks, 2) pressure-sensitive, laminating, and cold-foil adhesives, 3) cast and cure processes, and 4) overprint varnishes, primers, and many special-effects coatings. The only caveat is that LED-formulated UVA and UVV chemistry be used instead of chemistry originally designed for broad-spectrum output. Fortunately, many companies now offer dual-cure chemistry. Dual-cure formulations are specifically engineered for LED lamps but include further adjustments that also make them suitable for mercury-vapor lamps. This reduces the number of SKUs and eases the transition of companies running a single line or multiple lines with both types of curing lamps.
Another important consideration is that the amount of energy emitted by UV-LED curing systems must be enough to deliver full cure at the intended line speed. Not all LED systems at the same wavelength (nm) and peak irradiance (Watts/cm2) emit the same total energy (Joules/cm2). When properly matched and integrated, narrow-web converting lines equipped with LED units consistently cure between 500 and 1,000 fpm (150 and 300 mpm). An increasing number of mid- and wide-web converting lines, particularly those running inks, are actually pushing speeds between 1,000 and 2,000 fpm (200 and 600 mpm). These faster speeds are possible only with correctly sourced and integrated LED lamps paired with suitable chemistry and integration.
When it comes to more functional formulations such as hardcoats, clearcoats, topcoats, silicone-release, COF coatings, and hot-melt adhesives, UV-LED curing will eventually be an option, but most applications are not yet technically or economically feasible. Remaining challenges generally include reformulating this category of more-demanding chemistry, potentially creating new molecules, developing UV-LED emitting systems with shorter UVB and UVC wavelengths to cure chemistry that will not react to UVA, and the time and resources necessary to demonstrate capabilities and optimize processes directly on existing narrow-, mid-, and wide-web manufacturing lines. Fortunately, many UV-coating suppliers are actively engaged in developing LED solutions for these remaining functional formulations and hope to bring new offerings to market soon.
One of the biggest challenges continues to be that mercury-vapor lamps and UV-LEDs do not emit the same spectral output and, therefore, are not a direct swap without changing chemistry. Most functional formulations were initially designed to react to shorter UVC wavelengths around 254 nm to produce the necessary final-cure properties. Unlike most inks, varnishes, and adhesives, many functional chemistry formulations simply do not react to longer UVA and UVV wavelengths. When they are reformulated to react to UVA and UVV, many of the desired functional characteristics are sometimes lost or result in other non-desired effects. For example, chemistry that is designed to react to longer UVA and UVV wavelengths sometimes yellows or clouds. For inks and graphic coatings, this discoloration can be masked through pigments or innovatively blending reactive ingredients. The effectiveness of these tactics, however, is less viable with industrial hardcoats and clearcoats that require full optical transparency.
Where suitable chemistry has been developed to work with commercial UV-LED curing systems, market traction has materialized. Where viable chemistry does not yet exist, development activity is heavily dependent on converters communicating strong interest in LED formulations to suppliers who must then allocate resources to development. Fortunately, shorter-wavelength LEDs are being developed by semiconductor fabricators and may be necessary in a few cases. These UVC-LED offerings currently exist as expensive prototype solutions not yet economically or technically viable for most manufacturing processes. Output from UVC devices remains low compared to UVA and is limited to a few non-ideal wavelengths. Source-life and efficiencies also are currently poor.
Conclusions
The good news is that the overriding market potential of UV-LED curing, increasing engagement by potential users, and steady adoption by converters is more firmly established with each passing year. This is concrete evidence that the present and future of web converting most certainly includes LED UV technology. As a result, converters are highly encouraged to speak with formulators regularly on the status of LED chemistry specific to their applications and express interest in ongoing development of functional materials. UV-LED curing system suppliers also should be included in those discussions. This is because LED UV-curing development and successful integration into manufacturing lines is an iterative process. It includes education, collaboration among suppliers and converters, pilot- and manufacturing-line trials, trial feedback that further drives formulation and LED-system optimization, integration, shifting converting jobs to LED, and ongoing learning. Consistent engagement across the supply chain enables web converters to become more aware of what is possible today; provide justification for LED retrofits and new LED lines; drive necessary innovation in the remaining functional formulation categories; and ultimately deliver the operation, economic, performance, and sustainability benefits naturally inherent with UV-LED curing.
Jennifer Heathcote, business development mgr. at GEW, Inc. (North Royalton, OH), holds a Bachelor of Science in Mechanical Engineering from Purdue University and an MBA from the Fisher College of Business at The Ohio State University. A subject matter expert on mercury-vapor and LED UV-curing systems as well as their use across a broad range of graphic-arts, product-decorating, and industrial applications, her knowledge spans digital inkjet and analog printing, PSAs and structural]-bonding adhesives, and industrial coating, converting, and finishing. Jennifer is active in RadTech North America, the Assn. for Ultraviolet and Electron Beam Technologies, and has served on its executive board. She regularly presents at industry conferences, events, and webinars, has authored over two dozen technical and commercial papers on UV curing, and in 2012, she co-authored The UV LED Handbook. Jennifer can be reached 440-237-4439, jheathcote@gewuv.com, at linkedin.com/in/Jennifer-Heathcote/, www.gewuv.com
Comments