By Mark Miller, CEO, Coating Tech Slot Dies LLC
The fluid coating world is one of steady state flow and continuous control. This world collides with the discrete coating needs of intermittent coating for products in the energy sector. To succeed in modern intermittent coating via slot-die technology for lithium-ion (Li-ion) battery technology, the rheology of the fluid needs to be understood and the process needs to be precise and in control. This is both a technology and economic concern for the battery industry. The line speed, valve speed and slot-die movement momentum all play into the ability to coat quickly and precisely.
Editor’s Note: This technical paper is based on a Battery Day keynote presentation at the AIMCAL R2R USA Virtual Conference held in October 2020.
Introduction
Fluid control and physical movement need to be investigated and understood to have success in coating rectangular patterns. Use of both seem to create the best effects for reduced head/tail development, reduced edge bead, and improved parallel-edge effects when dealing with the slurry viscosities in the 2,000- to 5,000-cP range of lithium-ion (Li-ion) battery cathode and anode slurries. Surface modification and vacuum were not considered as helpful in the past but need to be considered as important options in the future. Increased line speeds will be required to improve performance and reduce costs.
In this ever-progressing field, developing a deeper understanding of raw-material rheology and process limitations provide the framework for new developments and breakthroughs in the intermittent-coating industries. Investigations into line-speed limitations, die swell (liquid spread) and the effect of forces (gravity, pressure, viscosity and surface energy) are investigated in this area of research. Intermittent coating continues to be an important and growing area in the world of slot-die coating technology in general, and the breakthrough flexible-electronics and energy-storage industries in particular.
Any [rectangular] shape can be coated
Every coating process has a start and stop. In intermittent patch coating, this occurs more often than at the beginning and end of a roll. Of course, the steady-state continuous flow in between starting and stopping has been discussed at length and has its own issues to deal with. But what special issues are associated with the start and stop of a coating head? For this discussion we will concentrate on slot-die patch coating. Any shape can be coated – as long as it is a rectangle!
In the startup and stop flow analysis, the challenge is to reduce waste and defects associated with the transition from fluid flow to not flowing. The considerations of startup include wetting of the fluid on the substrate, pump control of fluid dynamics, and physical position of the coating equipment to the substrate.
Wetting: The ability of the fluid to displace air at the fluid/solid interface in coating is critical to reducing defects. If the surface tension of the fluid and the surface energy of the substrate are not compatible, the start of the coating bead may be delayed or jagged. This would lead to an improper “head” of the coated patch. Surface-energy modification may be required to produce a solid patch (see previous technical paper on page 00).
Pump Control: There are many techniques for intermittent coating, with the most widespread technique using valve control of the fluid and physical movement of the coating head. The valve acts as the immediate start/stop of fluid flow, while the mechanical movement of the coating head breaks the wetted bead. The timing of the valve control with the mechanical movement can produce a good head or a poor start that includes poor bridging of the fluid, streaks, or a heavy “head.”
Physical Position: Where the coating head sits in relation to the substrate can determine the output flow of the fluid “head.” As a liquid surface approaches a substrate, the liquid boundary layer has vapor molecules that begin to adsorb to the substrate surface. This adsorption forms a bridge when the concentration is high enough. If the bridge occurs with a concentration that is even across the coating width, the coating will be uniform for the “head” or beginning of the coated patch. If the concentrated vapor is too far away, the substrate surface too rough, or the concentration of the fluid fluctuates, the coating will create a curved coating “head” to the patch.
The considerations of stopping are similar to startup but need to consider fluid reaction to mechanical and rheologic behavior. The resultant “tail” of the coated patch is a function of the timing between the valve for fluid control, mechanical movement of the coating head, and wettability of the substrate by the fluid.
There is not just one way to coat patches of fluid onto a substrate, but as long as you are aware of the coating fundamentals, you will be successful in your intermittent-coating operation.
Line-speed experiment on patch coating
In this experiment of intermittent coating, the goal was to see the main effects of increased line speed. Faster fluid flow and higher line speeds change the physics of the start-and-stop phenomena, regardless of the valve-reaction times and the geometries of the equipment involved.
Intermittent coating (or patch coating) is coating with a “start and stop” shorter than the length of the full roll and defined by an uncoated border. In the case of slot-die intermittent coating, the shape is always a rectangle. Intermittent coating can be completed in full web or lane coating, creating rectangles down-web from the coating source. The limit is based off patch control and reaction time.
Why would someone be interested in coating a discrete patch of coating instead of full-web continuous coating? Money. The most common example is in the world of battery-component substrate coating. When coating an anode or cathode, fuel cells and Li-ion batteries require an uncoated border to reduce the chance of a short circuit in the final construction. What this experiment was designed to evaluate is the limits of the coating width and spacing allowable with slot-die coating technology. The application of Li-ion battery technology, along with the product specifications, provided the outline for the experimental procedure and conclusions.
Patents: One of the earliest known patents regarding slot-die technology and intermittent coating is credited to Edward Choinski (US Patent 4,938,994) from 1990. This describes the basis of intermittent coating required for printed electronics based off flow control and mechanical movement. It also describes multilayer fluid coating to allow for more than one fluid to be coated simultaneously on the same side of the substrate.
Further information (and the patent closest to the current arrangement) is described by a 3M Co. patent (US Patent 5,360,629), where a pattern of discrete patches is spaced on a moving substrate. These coatings are created with a slot die and metering pump where a valve directs the fluid to either the slot die or a holding tank. The three-way valves are important to most intermittent-coating applications today. Description of the PLC control provides insight into the process for in this experiment.
Another patent application from Watanabe, et al. (US Patent 5,824,156) describes a physical reduction in flow (shut-off bar positioned within the slot die) to eliminate flow through a position within the slot opening. This shut-off bar is located internal to the slot die, while some non-patented applications have considered a shut-off bar external to the slot opening.
Specifications: For lithium-ion battery applications, the following data set is common, and was the basis for the experiment.
1. Coating width = 340 mm
2. Coating length = 680 mm
3. Intermittent-coating capability required up to 10, 20 and 30 mpm with maximum 3-mm tail allowed
4. Cross-web variation tolerance based off weight (5 +/-0.15 mg/cm2)
5. Required coating speed: at least 10 mpm for a slurry with loading of 6 mg/cm2 and 50% solids.
6. Coating-edge quality to match cross-web coating variation capability
7. Tension-control improvement to match down-web coating variation capability based off weight (5 +/-0.15 mg/cm2).
8. Multiple coating stations considered
Head and tail development: The initial start and final stop flow control is what is considered the “head” and “tail” of an intermittently coated patch. Following industry protocol, the goal was to reduce this increased or decreased thickness to a maximum of 3 mm in length when coating a discrete patch at 10 mpm. The head and tail occur for reasons of typical start-and stop phenomenon (cross-web caliper control, velocity gradient, pressure gradient, and volumetric flow).
Edge effects: When the anode or cathode is coated onto a foil (aluminum and copper, respectively), besides the start-and-stop phenomenon of the head and tail, we also have to deal with edge effects. When assembling the final battery cell, any significant variation in coatweight will cause issues in the battery performance. Edge effects occur for three main reasons: surface tension, film stretching, and die swell.
The combined head and tail effects with edge effects may seem small in the coated and calendered product, but the stack of multiple layers can increase the variation by millimeters and cause unevenness, wrinkling, folding, and power differentials – none of which are allowable in energy-storage devices. This current distribution variation is recognized, in the worst case, as a spike in energy with run-away heat. Heavy edges increase battery-cell volume and decrease energy density.
Patch control
There are two fundamental methods for creating discrete coatings on continuous webs: flow control and mechanical movement. The response time of each is critical to the resulting patch quality. This is the realm of computer control, electrical response and programing know-how. The intermittent programing logic needs to control as much as the die positioner movement, valve start and stop, web control, and possibly vacuum for fluid pinning. The response time of the valve and the time required for physical movement are the controlling factors of the patch length.
Flow control: In a typical arrangement, a 3-way valve controls the flow of fluid toward and away from the slot die. Flow control includes valves that work within the closed slot-die system to allow for flow that is diverted when not needed for coating, and shut-off bars within and external to the slot die to keep internal fluid flow within the slot die from escaping (see Figure 1).
FIGURE 1. In a typical arrangement, a 3-way valve controls the flow of fluid toward and away from the slot die.
Mechanical movement: If the fluid flow control is not enough to break the bead, then mechanical movement of the equipment is required to create clean starts and stops for the rectilinear patch. The mechanical movement can include moving the slot die away from the substrate or moving the substrate away from the slot die. This movement can be controlled by pistons that react with air or mechanical positioning. Another option is to use a cam for controlled motion with valving to match the cam motion.
Experimental
Water-based anode and NMP-based cathode slurries were used with aluminum and copper substrates for coating understanding. These raw materials are typical for Li-ion battery applications. Rheological data shows shear thinning behavior for both fluids across the shear-rate range experienced in slot-die coating (1-5,000 sec-1) (see Figure 2).
After anode and cathode were coated, cut-outs and cross sections were taken and analyzed to determine the relative heights to the average cross-web profile. These are final dried profiles, and not wet.
The slot die was of uniform dimensions for all applications:
· Offset = 0
· Lip face (upstream and downstream) = 762 microns (0.030 in.)
· Shim thickness = 500 microns (20 mil)
· Coating gap = 25 microns (1 mil)
· Coating width = 340 mm (13.38 in.)
Results and discussion
It is damaging to the final battery-cell assembly if the head, tail, or edges of coating differ in height in comparison to the bulk of the coated anode or cathode slurry. In addition to height variation, the width of the unwanted bead and the parallel nature of the coated line is important.
What was found in experimental results was that the parallel line is a function of line speed. As line speed increased, the parallel nature was less difficult to maintain. This is a combination of the wetting effects of the shear-thinning fluid and the reduced pulsation at the higher line speeds.
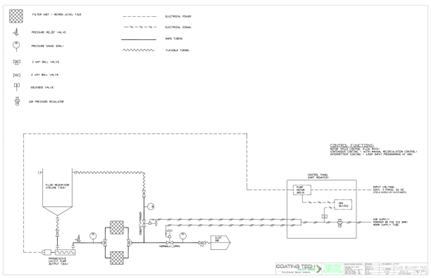
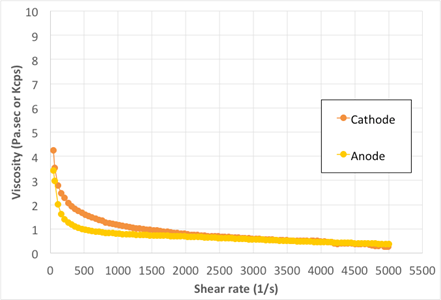
FIGURE 2. Rheological data shows shear thinning behavior for both fluids across the shear-rate range experienced in slot-die coating.
Another important phenomenon is the effect of obtaining steady-state flow conditions for the fluid prior to engaging the slot die. The critical concern is making sure the flow rate is smooth at the exit and air is not trapped in the system. In the “head” of the coating, if there is a pressure overshoot, then the fluid comes out at an increased velocity. Even if the pressure is stabilized, air in the system will allow the fluid to flow excessively. Measuring and balancing pressure within the slot-die valve system improved head and tail development.
In edge effects, whether considering surface tension, film stretching or die swell, stresses are imparted in the fluid from the slot die. These stresses develop a draw ratio D, which is defined as a ratio of the substrate speed (vsubstrate) to the fluid speed (vfluid). The edge height is taller by a factor of the draw ratio (D1/2). Proper mechanical design of the slot die for the rheology and similar fluid-to-substrate speed improved edge effects.
In the second set of experiments, the line speed was increased from 10 mpm to 20 mpm and finally to 30 mpm. The resultant effects were associated with these speed increases. Parallelism was affected by line speed and investigation of vacuum to reduce these effects would be the subject of further study. The test at 20 mpm did not push the product out of specification, but it was difficult to maintain a 30-mpm parallel product.
The addition of a second coating station for a portion of the trials allowed for some improvement in coating performance, but the line-speed to fluid-flow draw ratio was still important to final product performance.
Sensitivity to air and pressure variations increased with increased line speeds. Reduction of connections along the flow path in the fluid-delivery system helped reduce the number of areas for air infiltration. Monitoring the pressure on each leg of the coating and recirculation path also was more important. Addition of flow monitoring may have added benefit to pressure control in the fluid-delivery system, but this would add more connections for air infiltration.
The final new finding was in lot-to-lot variability of the rheology of the fluid. When coating the same fluid, but a different lot of material, the results may shift without process shifts. This seemed to be attributable to the variation in viscosity versus shear-rate behavior of the slurry lots. It is suggested to monitor and log these variations as studies continue.
Conclusions
Intermittent coating continues to be an important and growing field in the world of slot-die coating technology in general, and the flexible-electronics and energy-storage industries in particular.
With lithium-ion battery-coating applications, the ability to meet the current production requirements for a simultaneous, dual-sided, intermittently coated anode and cathode is possible in the 10- to 30-mpm range. The question is where the upper limit is and what advances need to be developed for higher production speeds and improved energy density.
Fluid control and physical movement were investigated for this experiment. Use of both seemed to create the best effects for reduced head/tail development, reduced edge bead, and improved parallel edge effects. Surface modification and vacuum were not considered for this work. Developing a deeper understanding of raw-material rheology, experiments with process limitations will provide the framework for new developments and breakthroughs in the intermittent-coating industries.
References
1. Kistler, S.F., Schweizer, P.M., Liquid Film Coating: Scientific Principles and Their Technological Implications. Chapman & Hall, New York (1997).
2. Gutoff, E.B., Cohen, E.D., Kheboian, G.I., Coating and Drying Defects: Troubleshooting Operating Problems. Wiley-Inter-science, New York (2006).
3. Choinski, E.J., 1990, “Method and apparatus for patch coating printed circuit boards,” US Patent 4,938,994.
4. Milbourn, T.M. and Barth, J.J., 1994, “Method of applying discrete coating patches to a moving web,” US Patent 5,360,629.
5. M. Watanabe, Y. Ueyama, T. Nakamura, Y. Ohana, T. Hayashi, 1998, “Intermittent coating apparatus, intermittent coating method and manufacturing method of battery electrodes, and non-aqueous electrolyte cell,” US Patent 5,824,156.
Mark Miller, CEO of Coating Tech Slot Dies LLC (Eau Claire, WI), holds a Bachelor’s degree in Chemical Engineering from the University of Wisconsin-Madison, a Master’s degree in Polymer Science and Technology from Lehigh University, and a J.D. from Hamline Mitchell School of Law. He has worked at 3M Co., and Coating Tech Slot Dies, and is a frequent presenter at AIMCAL R2R Conferences. Mark can be reached at 715-456-9545, mark.miller@slotdies.com, www.slotdies.com
Comments